How Batteries Work, What Types Exist, and Why Their Performance Matters
In today’s technology-led world, batteries are everywhere, serving as key carriers of energy storage and conversion. From everyday smartphones and laptops to electric vehicles and grid-level energy storage, batteries support the functioning of diverse systems and equipment. Understanding battery basics is essential for efficient energy use, technological innovation, and solving challenges in the energy sector.
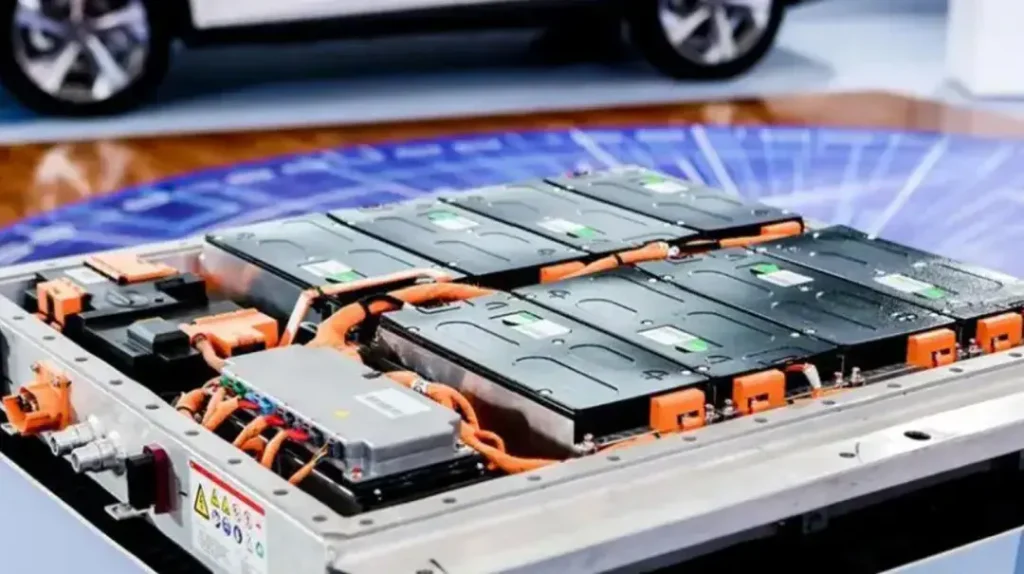
1. Electrochemical Principles of Batteries
(1) Electrode Reactions and Ion Migration
At the heart of battery basics lies electrochemical reactions and ion migration. Take lithium-ion batteries as an example: they contain a cathode and an anode, separated by an electrolyte. During charging, lithium ions (Li⁺) are deintercalated from the cathode due to oxidation and migrate through the electrolyte to the anode, while electrons travel via the external circuit to the anode. At the anode, lithium ions are intercalated into the anode material’s lattice via a reduction reaction. During discharge, the process reverses—lithium ions return to the cathode, and electrons power external devices via the external circuit.
In lead-acid batteries, the chemistry is more complex. The anode is lead (Pb), the cathode is lead dioxide (PbO₂), and the electrolyte is a sulfuric acid (H₂SO₄) solution. During charging, lead sulfate (PbSO₄) at the anode is reduced to lead, and at the cathode, PbSO₄ reacts with water to form PbO₂, releasing sulfate (SO₄²⁻) and hydrogen ions (H⁺). During discharge, Pb is oxidized back to PbSO₄, and PbO₂ reacts with sulfuric acid to form PbSO₄ and water. Sulfate ions migrate within the electrolyte to maintain charge balance.
(2) Chemical Changes During Charging and Discharging
Different types of batteries undergo different chemical changes. A clear understanding of these processes is essential to mastering battery basics. In lithium-ion batteries with LiCoO₂ cathodes, lithium ions deintercalate during charging, increasing Co’s oxidation state from +3 to +4. At the anode, lithium ions intercalate into graphite to form LiₓC₆. With LiFePO₄ cathodes, charging raises Fe’s oxidation state from +2 to +3. These reactions determine differences in energy density, cycle life, and safety.
In NiMH batteries, the cathode is nickel hydroxide (Ni(OH)₂), and the anode is a hydrogen-absorbing alloy (M). Charging oxidizes Ni(OH)₂ to NiOOH and generates OH⁻ ions. The anode forms metal hydrides (MH). During discharge, NiOOH is reduced back to Ni(OH)₂, and the hydrides release hydrogen and electrons.
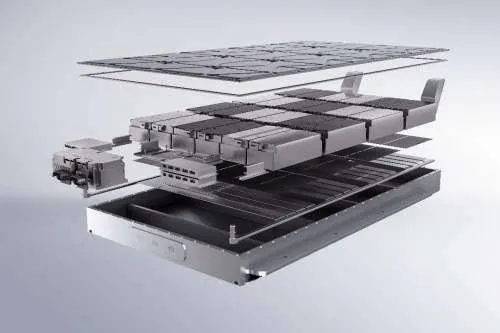
2. Battery Types and Characteristics
An essential part of battery basics is knowing the differences between battery types and their suitability for different applications.
(1) Lead-Acid Batteries
Features and Pros/Cons: Lead-acid batteries are traditional and low-cost, with mature technology and good safety. They offer high instantaneous discharge currents, making them ideal for starting automotive engines. However, they have low energy density, short cycle life (300–500 cycles), and contain toxic lead, posing environmental risks.
Applications: Common in low-speed EVs, backup power systems, and solar streetlights. Their high discharge current makes them suitable for engine starting.
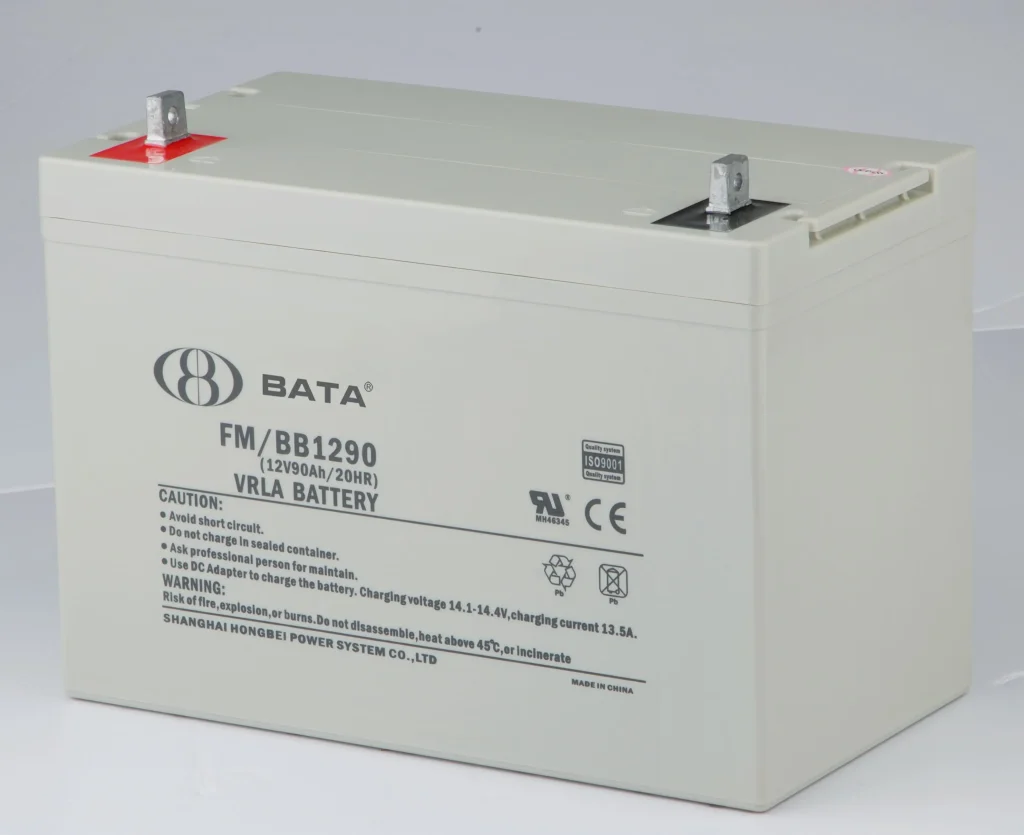
(2) Lithium-Ion Batteries
Performance Differences by Material:
- LiCoO₂: High energy density, suitable for portable electronics, but expensive, scarce, and relatively unsafe.
- LiFePO₄: Excellent safety and thermal stability, over 2000 cycles, but lower energy density; used in energy storage, tools, and low-speed EVs.
- Ternary (NCM/NCA): Balanced energy and performance; high-nickel versions boost density but reduce thermal stability.
Applications: Widely used in consumer electronics and EVs. Ternary batteries power premium EVs, while LiFePO₄ suits cost-sensitive, safety-prioritized models. They are also becoming mainstream in energy storage projects.
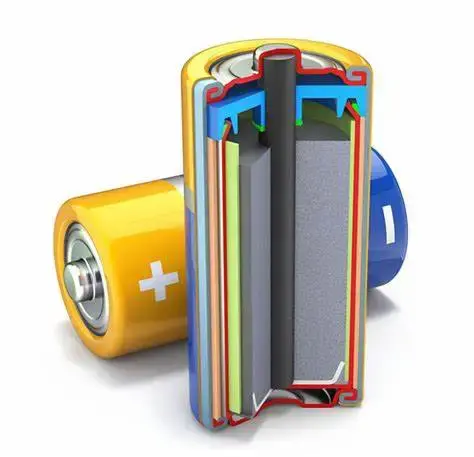
(3) NiMH Batteries
Features and Pros/Cons: NiMH batteries have high charge efficiency, fast charging, and overcharge resistance. More eco-friendly than NiCd batteries. Downsides include lower energy density and high self-discharge.
Applications: Used in digital devices and hybrid vehicles, NiMH batteries contribute to energy recovery and stable performance.
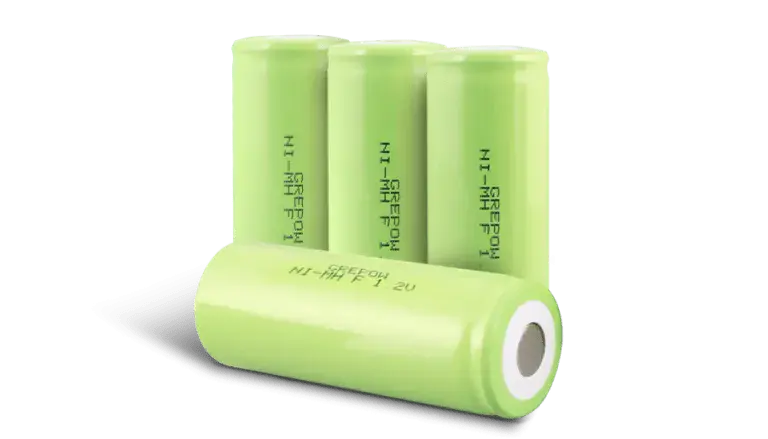
3. Battery Performance Parameters
Battery basics also include knowing how to evaluate a battery’s performance. Key metrics include:
(1) Capacity
Capacity is the total charge a battery delivers under specified conditions, measured in Ah or mAh. A 10Ah battery can discharge at 1A for 10 hours. It’s affected by materials, discharge current, temperature, and aging. Cold reduces ion mobility and reaction rates; heat accelerates material aging. Battery capacity declines as materials degrade with cycles.
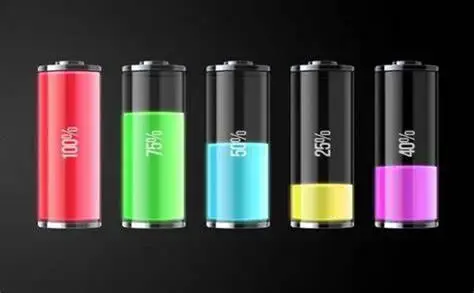
(2) Voltage
Voltage is the potential difference between terminals, measured in volts (V). Each battery type has a nominal voltage (e.g., 3.7V for lithium-ion). Real-world voltage fluctuates during operation. Stable voltage ensures reliable device performance. Open-circuit voltage correlates with state of charge (SOC). In battery packs, voltage consistency between cells is crucial to performance and lifespan.
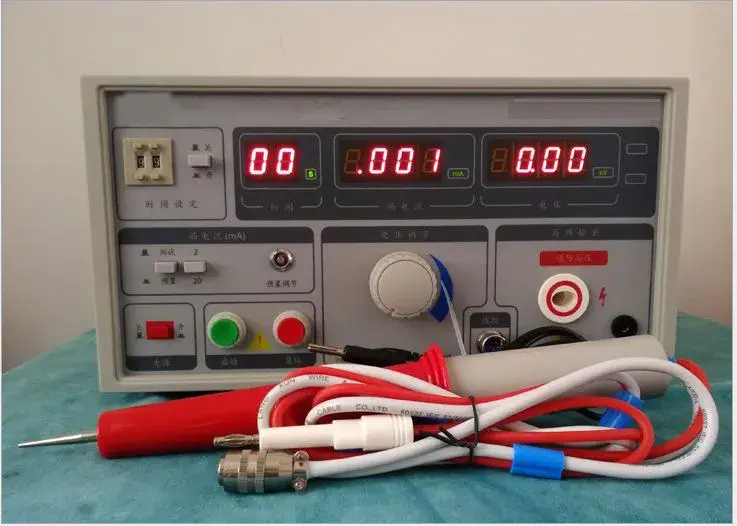
(3) Internal Resistance
Internal resistance includes ohmic and polarization resistance. It is measured via AC impedance or DC discharge. High resistance leads to energy loss, heat generation, and power decline. It increases with aging, becoming a key indicator of performance deterioration.
(4) Charge/Discharge Efficiency
Efficiency = (energy stored or released) ÷ (input or stored energy). Li-ion batteries have 90–95% efficiency; lead-acid: 80–90%. Influenced by battery type, current, temperature, and aging. Large currents and extreme temperatures reduce efficiency. As batteries age, efficiency drops due to side reactions and material degradation.
(5) Cycle Life
Defined as the number of cycles until capacity drops to 80% of the original. EVs require 1000–3000 cycles; storage batteries need even more. Affected by battery type, current, temperature, and BMS usage. Proper charging, moderate current, and temperature control extend life. BMS monitors key metrics and protects from overcharging/discharging.
(6) Self-Discharge Rate
Self-discharge refers to idle energy loss from spontaneous reactions. Measured as monthly capacity drop. NiMH: 10–30%; Li-ion: 2–5%. High temperature accelerates discharge. High-purity materials and quality manufacturing reduce it. Cool, dry storage and periodic recharging help preserve battery health.
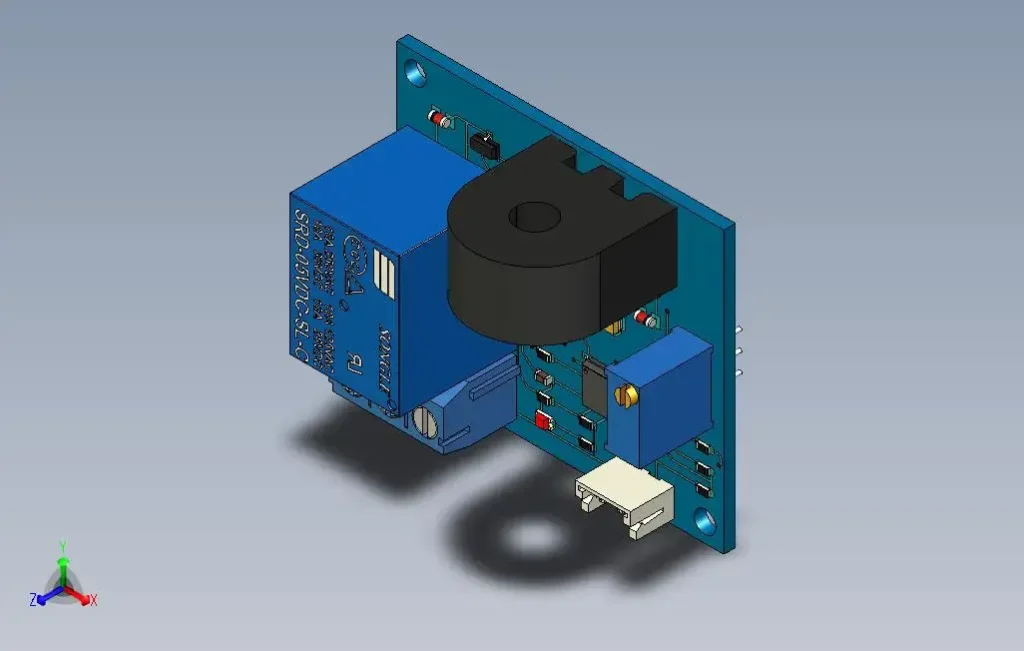
Conclusion
Understanding the electrochemical principles, types, and performance metrics of batteries is fundamental to battery basics. This knowledge empowers users to make informed decisions, maximize battery performance, and support innovation. As energy demand and environmental awareness grow, battery technology will continue to evolve—anchored by the foundational concepts of battery basics.